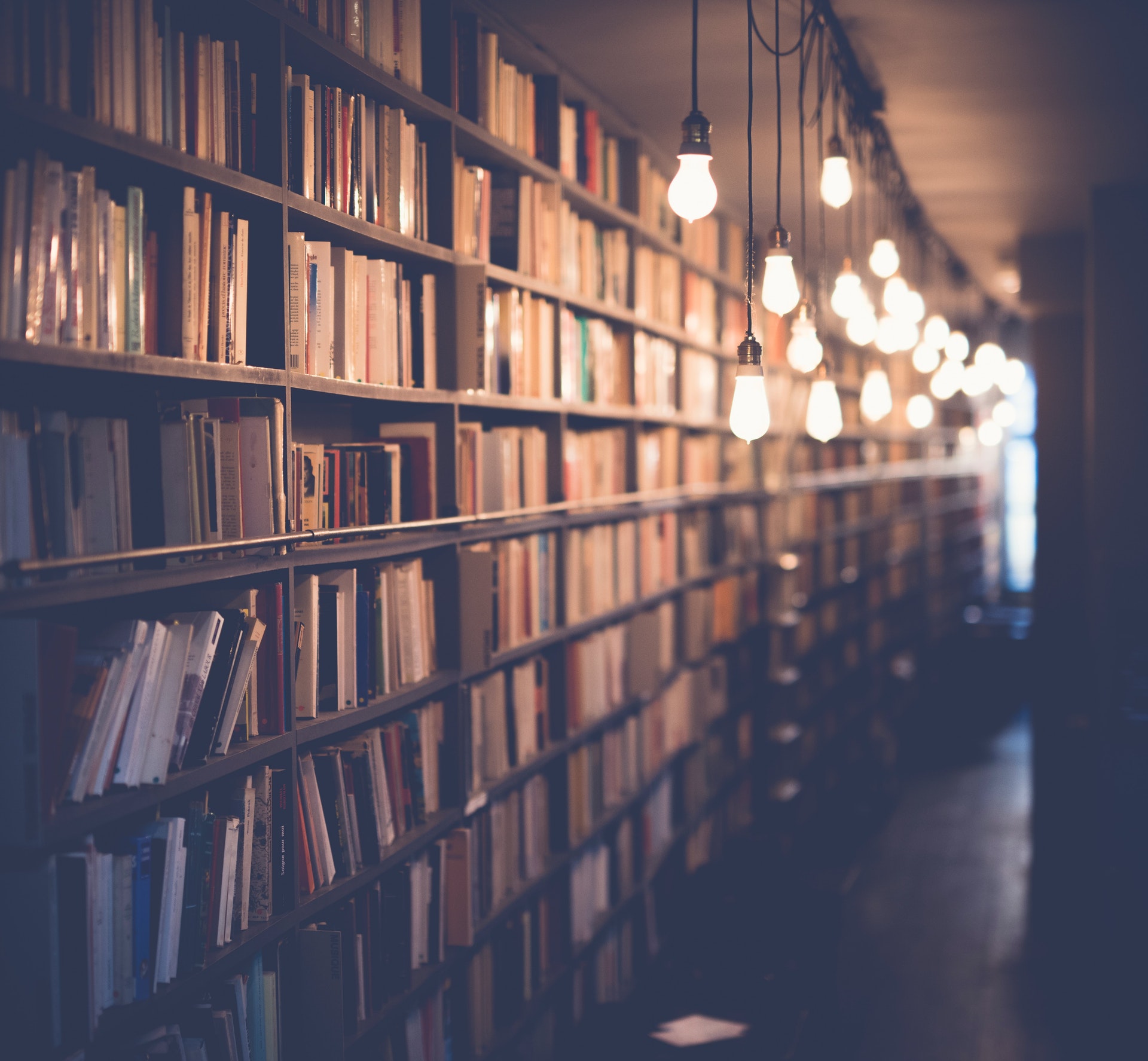
There are numerous types of receptor potentials, each with their own set of associated physical and chemical properties. As a result, it is difficult to make any definitive claims about receptor potentials as a whole. That being said, it is possible to make some claims about certain types of receptor potentials. For instance, it is false to say that all receptor potentials result in the opening of ion channels. In fact, some receptor potentials actually result in the closing of ion channels. Additionally, not all receptor potentials are graded; some are all-or-none. Finally, receptor potentials do not always lead to the activation of cell signaling pathways; sometimes they can actually lead to the inhibition of cell signaling pathways.
Intriguing read: What Is Friction?
Receptor potentials are graded potentials that occur in response to a stimulus.
When a neuron receives a stimuli, it will create a graded potential in the post synaptic membrane in order to send an electrical signal down the axon. The graded potential will be generated by the opening and closing of ion channels in the membrane. The ion channels will be opened in response to the stimuli, which will cause an influx of ions into the cell. This will change the membrane potential, which will generate an electrical signal that will travel down the axon. The amplitude of the graded potential will depend on the intensity of the stimuli.
Recommended read: Can You Use Bleach on Your Areola?
Receptor potentials are all-or-none potentials.
In its simplest form, the all-or-none law states that the strength of a muscle contraction is the same regardless of the strength of the stimulus that triggered it. This law applies to cardiac muscle and smooth muscle in the walls of blood vessels and organs like the stomach, as well as to skeletal muscle. It is also the basis for the action potential, the nerve impulse that transmits information from the central nervous system to the muscles.
The all-or-none law has two parts. The first part states that the strength of a muscle contraction is directly proportional to the number of motor units that are activated. A motor unit is a nerve cell and the muscle fibers it innervates. The second part of the all-or-none law states that all of the muscle fibers in a motor unit will contract when the unit is stimulated, but they will all relax when the stimulation stops.
The all-or-none law is an important principle in physiology because it ensures that the strength of muscle contractions is consistent and predictable. It also helps to protect the body from damage by preventing muscle fibers from being over-stimulated and contracting too forcefully.
The all-or-none law is not absolute, however. There are a few situations in which the strength of a muscle contraction can vary, even when the number of motor units activated is the same.
One situation in which the all-or-none law does not apply is when muscles are tired. When muscles are fatigued, they produce less force and the all-or-none law no longer holds true.
Another situation in which the all-or-none law does not apply is when muscles are stimulated by electrical stimuli of different intensities. When muscles are stimulated by weak electrical stimuli, they produce small, brief contractions known as twitches. If the electrical stimulus is strong enough, it can override the all-or-none law and cause the muscle to contract with greater force than it would if all of the motor units were activated.
The all-or-none law is a important principle in physiology that helps to ensure that muscle contractions are consistent and predictable. However, there are a few situations in which the all-or-none law does not apply.
Broaden your view: What Are the Best Places to Elope in California?
Receptor potentials can be either excitatory or inhibitory.
In a healthy adult human, the central nervous system relies on a balance of excitatory and inhibitory neurotransmission to maintain normal function. Excitatory neurotransmission promotes action potential firing and promotes the transmission of signals from one neuron to the next, while inhibitory neurotransmission suppresses action potential firing and limits the transmission of signals from one neuron to the next.
Receptor potentials are the changes in membrane potential that occur when a neurotransmitter binds to its receptor. These changes can be either excitatory or inhibitory.
Excitatory receptor potentials lead to an increase in membrane potential, which makes it more likely that an action potential will be generated. Inhibitory receptor potentials lead to a decrease in membrane potential, which makes it less likely that an action potential will be generated.
The balance of excitatory and inhibitory neurotransmission is important for normal neuronal function. Too much excitatory neurotransmission can lead to excessive action potential firing, which can lead to seizure activity. Too much inhibitory neurotransmission can lead to reduced action potential firing, which can lead to depression and other mental health disorders.
Receptor potentials can have a significant impact on the function of the central nervous system. It is important to understand the role that they play in order to maintain a healthy balance of excitatory and inhibitory neurotransmission.
Explore further: Wolves Maintain Homeostasis
The size of a receptor potential is determined by the intensity of the stimulus.
A receptor potential (RP) is a change in the membrane potential of a cell that occurs in response to a stimulus. The size of an RP is determined by the intensity of the stimulus, as well as the number and type of receptors present. The intensity of the stimulus determines the number of receptors that are activated and, consequently, the size of the RP. The number of receptors present determines the amplitude of the RP. The type of receptor influences the duration of the RP.
Receptor potentials were first described in the late 19th century by German physiologist Ernst Weber. Weber observed that the electrical potentials generated by sensory stimuli varied in both magnitude and duration. He suggested that these differences were due to the different number and types of receptors that were activated by the different stimuli.
The size of an RP is affected by both the intensity of the stimulus and the number of receptors activated. The greater the intensity of the stimulus, the greater the number of receptors activated and, consequently, the larger the RP. The number of receptors present also affects the size of the RP. The more receptors that are present, the greater the amplitude of the RP.
The type of receptor also influences the duration of the RP. Excitatory receptors, such as those for touch, heat, and pain, result in an RP that lasts for a short period of time. Inhibitory receptors, such as those for pressure and cold, result in an RP that lasts for a longer period of time.
Receptor potentials are important for the encoding of information about stimuli in the nervous system. They provide a way for the nervous system to compare the intensity of different stimuli and to determine the appropriate response.
On a similar theme: Advertising Influences Consumer Choice
Receptor potentials can be summated.
The nervous system uses electrical signals to relay information between neurons. These electrical signals are transmitted by ions moving across the cell membrane. The cell membrane is made up of lipid molecules, which form a selectively permeable barrier that allows certain ions to pass through while keeping others out.
Ions are atoms that have gained or lost electrons, giving them a charge. The three main types of ions involved in nervous system function are sodium (Na+), potassium (K+), and calcium (Ca2+). Sodium and potassium are called “electrolytes” because they dissolve in water and conduct electricity. Calcium is a “divalent” ion, meaning it has two charges.
Neurons have special structures that allow them to selectively pump ions across their cell membranes, creating an “electrical gradient.” This gradient is essential for the proper function of neurons and the nervous system as a whole.
The cell membrane of a neuron has two types of proteins that serve as gateways for ions: “ion channels” and “pumps.” Ion channels are proteins that allow specific ions to cross the cell membrane. Pumps are proteins that use energy to move ions against the gradient.
Ion channels are either “voltage-gated” or “ligand-gated.” Voltage-gated ion channels are opened or closed by changes in the electrical potential across the cell membrane. Ligand-gated ion channels are opened or closed by “ligands,” small molecules that bind to the channel.
There are four major types of voltage-gated ion channels: sodium (Na+), potassium (K+), calcium (Ca2+), and chloride (Cl-). The most important voltage-gated ion channels for nervous system function are the Na+ channels and the K+ channels.
The Na+ channels are particularly important in the process of “action potentials.” An action potential is an electrical signal that is transmitted by neurons. This signal is generated by the opening and closing of Na+ channels in the cell membrane.
When the Na+ channels open, Na+ ions flow into the neuron. This influx of Na+ ions causes the inside of the neuron to become more “positive” with respect to the outside. This change in voltage is called “depolarization.”
A fresh viewpoint: Cancelling Turn Signals
Receptor potentials can lead to action potentials.
Receptor potentials are changes in the membrane potential of a cell that are caused by the binding of a chemical ligand to the cell's surface. These changes can lead to the generation of an action potential, which is a change in the membrane potential that propagates along the cell membrane.
Receptor potentials are typically found in sensory neurons, where they allow the cell to detect changes in the environment. For example, when a light is shone on the retina, receptor potentials are generated in the rods and cones of the eye. These potentials are then transmitted to the brain, where they are interpreted as a visual stimulus.
Receptor potentials can also be generated in response to touch, pressure, sound, and other stimuli. These potentials are then transmitted to the brain, where they are interpreted as sensations.
The binding of a ligand to a cell's surface can cause the generation of a receptor potential. However, not all ligands will produce a receptor potential. For example, some ligands bind to receptors that are not associated with a change in membrane potential.
Ligands that do produce receptor potentials typically bind to G-protein coupled receptors (GPCRs). GPCRs are a type of transmembrane protein that is found in the cell's membrane. When a ligand binds to a GPCR, it causes the GPCR to change conformation. This change in conformation activates a G-protein, which then activates enzymes that lead to a change in the cell's membrane potential.
The magnitude of the receptor potential that is generated by a ligand depends on the binding affinity of the ligand for the receptor, the number of receptors that are activated, and the number of G-proteins that are activated.
Receptor potentials can lead to the generation of an action potential. An action potential is a change in the membrane potential that propagates along the cell membrane. Action potentials are generated when the magnitude of the receptor potential exceeds a certain threshold.
When a receptor potential exceeds threshold, it causes an influx of ions into the cell. This influx of ions changes the membrane potential from negative to positive. This change in membrane potential causes an action potential to travel down the cell membrane.
The action potential then causes the release of neurotransmitters from the cell. Neurotransmitters are chemicals that allow communication between cells. They are released from the
Check this out: Gustatory Receptors
Receptor potentials are generated by ion channels.
Receptor potentials are generated by ion channels. These channels are responsible for the movement of ions, such as sodium and potassium, across the cell membrane. When these channels are open, ions are able to flow into and out of the cell. This flow of ions creates an electrical potential across the cell membrane, which is known as the receptor potential. The receptor potential is what allows nerves to communicate with each other and with other cells in the body.
Ion channels are found in all cells, but they are especially abundant in nerve cells. This is because nerve cells need to be able to send electrical signals quickly and efficiently. The speed at which an electrical signal can travel down a nerve cell is limited by the number of ion channels that are present in the cell. The more ion channels a cell has, the faster the electrical signal can travel.
There are two types of ion channels, voltage-gated and ligand-gated. Voltage-gated ion channels are opened or closed by changes in the electrical potential across the cell membrane. Ligand-gated ion channels are opened or closed by molecules that bind to them. These molecules are known as ligands.
The receptor potential is generated by voltage-gated ion channels. These channels are opened or closed by changes in the voltage across the cell membrane. When the voltage across the cell membrane changes, the ion channels will either open or close. If the cell is at rest, the ion channels will be closed. When the cell is stimulated, the voltage across the cell membrane will change and the ion channels will open. This change in voltage will cause an influx of ions into the cell, which will change the electrical potential inside the cell. This change in potential is known as the receptor potential.
The receptor potential is what allows nerves to communicate with each other and with other cells in the body. The receptor potential is generated by changes in the voltage across the cell membrane. These changes in voltage are caused by changes in the ion concentrations inside and outside the cell. When the ion concentrations inside and outside the cell are equal, the cell is at rest. When the ion concentrations inside and outside the cell are not equal, the cell is said to be depolarized.
Depolarization of a cell can be caused by many things, including changes in the concentration of ions outside the cell, changes in the concentration of ions inside the cell, or changes in the membrane potential. When
A fresh viewpoint: Highest Concentration
Receptor potentials are generated by G-protein coupled receptors.
In 1931, Otto Loewi discovered that nerve cells communicate with one another by releasing chemicals, which he called "transmitters". He showed that when the chemical acetylcholine was released from one nerve cell, it could stimulate another nerve cell to fire an action potential. This discovery revolutionized the field of neuroscience and led to the development of many different drugs that act by altering the levels of transmitters in the brain.
One important family of transmitters are the so-called "neurotransmitters", which include dopamine, serotonin, and norepinephrine. These transmitters are important for regulating mood, emotion, and cognition. Neurotransmitters are typically released from the presynaptic cell and bind to receptors on the post-synaptic cell. This binding triggers a change in the post-synaptic cell, which can lead to the activation of an action potential.
G-protein coupled receptors (GPCRs) are a type of receptor that is found in the cell membrane and is coupled to a G-protein. G-proteins are a type of protein that is found in the cell membrane and helps to transduce signals from the outside of the cell to the inside of the cell. GPCRs are important for many different cellular functions, including the regulation of neurotransmitter release.
One type of GPCR that is important for neurotransmitter release is the metabotropic glutamate receptor (mGluR). mGluRs are found in the brain and are important for regulating the release of neurotransmitters such as glutamate and GABA. Glutamate is an important excitatory neurotransmitter, while GABA is an important inhibitory neurotransmitter.
mGluRs are activated by the binding of glutamate to the receptor. This binding triggers a change in the G-protein, which leads to the activation of an enzyme called phospholipase C. Phospholipase C cleaves a molecule called phosphoinositol 4,5-bisphosphate (PIP2) into two molecules: inositol trisphosphate (IP3) and diacylglycerol (DAG).
IP3 binds to a type of receptor called an inositol trisphosphate receptor (IP3R), which is found on the endoplasmic reticulum. This binding triggers the release of Ca2+ from the endoplasmic ret
For more insights, see: Fix Drivetrain Binding
Receptor potentials can be depolarizing or hyperpolarizing.
Receptor potentials are changes in membrane potential that occur in response to the binding of a ligand to a receptor protein. These changes can be either depolarizing or hyperpolarizing, depending on the type of receptor and the type of ligand that binds to it.
Depolarizing receptor potentials occur when ligands that bind to excitatory receptors cause an increase in membrane potential. This increase in potential can depolarize the cell, making it more likely to fire an action potential. Excitatory ligands include neurotransmitters like glutamate and aspartate.
Hyperpolarizing receptor potentials occur when ligands that bind to inhibitory receptors cause a decrease in membrane potential. This decrease in potential can hyperpolarize the cell, making it less likely to fire an action potential. Inhibitory ligands include neurotransmitters like GABA and glycine.
Receptor potentials are important for mediating the effects of ligands on cell function. These potentials can influence the excitability of a cell and the strength of synaptic transmission.
You might like: Artificial Grass Decrease Home
Frequently Asked Questions
What is the function of receptor molecules in the brain?
Receptor molecules in the brain act as stimuli.
Which type of potential is carried by the neuroglia?
The receptor potential is carried by the neuroglia.
How do receptor potentials affect action potentials?
There are two types of receptor potentials: depressing and depolarizing.Depressing receptor potentials decrease the amplitude of an action potential, while depolarizing receptor potentials increase the amplitude of an action potential.
What is the action potential of a neuron?
The action potential of a neuron is an electrical signal that travels down the length of an axon in the form of a spike. This spike consists of a tiny amount of electricity and consists of three stages: the depolarization, the plateau, and the hyperpolarization.
What is the function of a neuron Quizlet?
A neuron's primary function is to relay information between different parts of the brain. Additionally, neurons play a significant role in controlling movement and sensation.
Sources
- https://en.lifeder.com/which-of-the-following-statements-about-receptor-potentials-is-false/
- https://www.timesmojo.com/can-a-neurotransmitter-be-both-excitatory-and-inhibitory/
- https://veasylife.com/which-of-the-following-statements-about-receptor-potentials-is-false/
- https://www.studocu.com/en-ca/document/the-university-of-british-columbia/introduction-to-biological-and-cognitive-psychology/week-5-discussion-answer/5262646
- https://theeducationlife.com/tag/which-of-the-following-statements-about-receptor-potentials-is-false/
- https://www.d.umn.edu/~jfitzake/Lectures/DMED/SensoryPhysiology/GeneralPrinciples/ReceptorPotential.html
- https://answers-all.com/science/what-causes-receptor-potential/
- https://medicalquiz.net/71891/
- https://setu.hedbergandson.com/are-graded-receptor-potentials-always-depolarizing
- https://estatename.com/which-of-the-following-statements-regarding-matter-is-false/
- https://knowitsdifference.com/what-is-the-difference-between-excitatory-and/
- https://knowledgeburrow.com/what-is-excitatory-and-inhibitory/
- https://quizlet.com/204755859/graded-receptor-potentials-and-action-potentials-flash-cards/
- https://www.faqsclear.com/are-receptor-potentials-graded/
Featured Images: pexels.com